The excitation-contraction coupling mechanism in skeletal muscle
- PMID: 28509964
- PMCID: PMC5425715
- DOI: 10.1007/s12551-013-0135-x
The excitation-contraction coupling mechanism in skeletal muscle
Abstract
First coined by Alexander Sandow in 1952, the term excitation-contraction coupling (ECC) describes the rapid communication between electrical events occurring in the plasma membrane of skeletal muscle fibres and Ca2+ release from the SR, which leads to contraction. The sequence of events in twitch skeletal muscle involves: (1) initiation and propagation of an action potential along the plasma membrane, (2) spread of the potential throughout the transverse tubule system (T-tubule system), (3) dihydropyridine receptors (DHPR)-mediated detection of changes in membrane potential, (4) allosteric interaction between DHPR and sarcoplasmic reticulum (SR) ryanodine receptors (RyR), (5) release of Ca2+ from the SR and transient increase of Ca2+ concentration in the myoplasm, (6) activation of the myoplasmic Ca2+ buffering system and the contractile apparatus, followed by (7) Ca2+ disappearance from the myoplasm mediated mainly by its reuptake by the SR through the SR Ca2+ adenosine triphosphatase (SERCA), and under several conditions movement to the mitochondria and extrusion by the Na+/Ca2+ exchanger (NCX). In this text, we review the basics of ECC in skeletal muscle and the techniques used to study it. Moreover, we highlight some recent advances and point out gaps in knowledge on particular issues related to ECC such as (1) DHPR-RyR molecular interaction, (2) differences regarding fibre types, (3) its alteration during muscle fatigue, (4) the role of mitochondria and store-operated Ca2+ entry in the general ECC sequence, (5) contractile potentiators, and (6) Ca2+ sparks.
Keywords: Ca2+ transients; Excitation–contraction coupling; Fibre types; Mitochondria; Skeletal muscle.
Figures
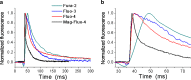
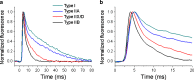
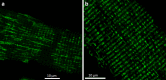
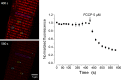
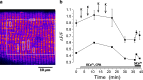
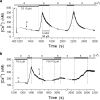
Similar articles
-
Excitation-contraction coupling in mammalian skeletal muscle: Blending old and last-decade research.Front Physiol. 2022 Sep 2;13:989796. doi: 10.3389/fphys.2022.989796. eCollection 2022. Front Physiol. 2022. PMID: 36117698 Free PMC article. Review.
-
A novel mechanism of tandem activation of ryanodine receptors by cytosolic and SR luminal Ca2+ during excitation-contraction coupling in atrial myocytes.J Physiol. 2017 Jun 15;595(12):3835-3845. doi: 10.1113/JP273611. Epub 2017 Feb 1. J Physiol. 2017. PMID: 28028837 Free PMC article.
-
Excitation-contraction coupling in skeletal muscle: recent progress and unanswered questions.Biophys Rev. 2020 Feb;12(1):143-153. doi: 10.1007/s12551-020-00610-x. Epub 2020 Jan 16. Biophys Rev. 2020. PMID: 31950344 Free PMC article. Review.
-
Preserved Ca2+ handling and excitation-contraction coupling in muscle fibres from diet-induced obese mice.Diabetologia. 2020 Nov;63(11):2471-2481. doi: 10.1007/s00125-020-05256-8. Epub 2020 Aug 25. Diabetologia. 2020. PMID: 32840676
-
Cannabinoid signalling inhibits sarcoplasmic Ca2+ release and regulates excitation-contraction coupling in mammalian skeletal muscle.J Physiol. 2016 Dec 15;594(24):7381-7398. doi: 10.1113/JP272449. Epub 2016 Oct 27. J Physiol. 2016. PMID: 27641745 Free PMC article.
Cited by
-
Ca2+-mediated coupling between neuromuscular junction and mitochondria in skeletal muscle.Neurosci Lett. 2021 May 29;754:135899. doi: 10.1016/j.neulet.2021.135899. Epub 2021 Apr 15. Neurosci Lett. 2021. PMID: 33865940 Free PMC article. Review.
-
Numb is required for optimal contraction of skeletal muscle.J Cachexia Sarcopenia Muscle. 2022 Feb;13(1):454-466. doi: 10.1002/jcsm.12907. Epub 2022 Jan 9. J Cachexia Sarcopenia Muscle. 2022. PMID: 35001540 Free PMC article.
-
Decrease in AQP4 expression level in atrophied skeletal muscles with innervation.Physiol Rep. 2021 May;9(9):e14856. doi: 10.14814/phy2.14856. Physiol Rep. 2021. PMID: 33991463 Free PMC article.
-
Sorafenib induces cachexia by impeding transcriptional signaling of the SET1/MLL complex on muscle-specific genes.iScience. 2024 Sep 10;27(10):110913. doi: 10.1016/j.isci.2024.110913. eCollection 2024 Oct 18. iScience. 2024. PMID: 39386761 Free PMC article.
-
Skeletal muscle metabolism and contraction performance regulation by teneurin C-terminal-associated peptide-1.Front Physiol. 2022 Nov 29;13:1031264. doi: 10.3389/fphys.2022.1031264. eCollection 2022. Front Physiol. 2022. PMID: 36523555 Free PMC article.
References
-
- Abbiss C, Laursen P. Models to explain fatigue during prolonged endurance cycling. Sports Med. 2005;35:865–898. - PubMed
-
- Adams B, Tanabe T, Mikami A, Numa S, Beam K. Intramembrane charge movement restored in dysgenic skeletal muscle by injection of dihydropyridine receptor cDNAs. Nature. 1990;346:569–572. - PubMed
Publication types
LinkOut - more resources
Full Text Sources
Other Literature Sources
Research Materials
Miscellaneous