Synthesis and modelling of the mechanical properties of Ag, Au and Cu nanowires
- PMID: 30956731
- PMCID: PMC6442207
- DOI: 10.1080/14686996.2019.1585145
Synthesis and modelling of the mechanical properties of Ag, Au and Cu nanowires
Abstract
The recent interest to nanotechnology aims not only at device miniaturisation, but also at understanding the effects of quantised structure in materials of reduced dimensions, which exhibit different properties from their bulk counterparts. In particular, quantised metal nanowires made of silver, gold or copper have attracted much attention owing to their unique intrinsic and extrinsic length-dependent mechanical properties. Here we review the current state of art and developments in these nanowires from synthesis to mechanical properties, which make them leading contenders for next-generation nanoelectromechanical systems. We also present theories of interatomic interaction in metallic nanowires, as well as challenges in their synthesis and simulation.
Keywords: 10 Engineering and Structural materials; 105 Low-Dimension (1D/2D) materials; 106 Metallic materials; 400 Modeling / Simulations; 500 Characterization, Nanomechanical Characterization; Coinage metal nanowires; molecular modelling; nanotechnology; synthesis method.
Figures
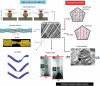
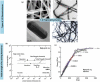
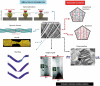
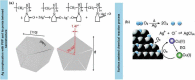
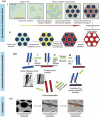
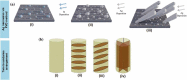
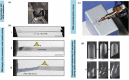
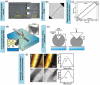
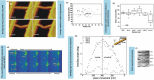
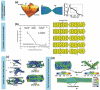
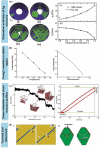
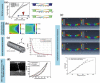
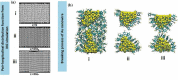
Similar articles
-
Using the hard templating method for the synthesis of metal-conducting polymer multi-segmented nanowires.Macromol Rapid Commun. 2011 Jan 3;32(1):25-34. doi: 10.1002/marc.201000486. Epub 2010 Oct 21. Macromol Rapid Commun. 2011. PMID: 21432967
-
Perovskite oxide nanowires: synthesis, property and structural characterization.J Nanosci Nanotechnol. 2010 Jul;10(7):4109-23. doi: 10.1166/jnn.2010.2942. J Nanosci Nanotechnol. 2010. PMID: 21128390
-
Multifunctional Metallic Nanowires in Advanced Building Applications.Materials (Basel). 2019 May 28;12(11):1731. doi: 10.3390/ma12111731. Materials (Basel). 2019. PMID: 31141962 Free PMC article. Review.
-
Complex-Morphology Metal-Based Nanostructures: Fabrication, Characterization, and Applications.Nanomaterials (Basel). 2016 Jun 6;6(6):110. doi: 10.3390/nano6060110. Nanomaterials (Basel). 2016. PMID: 28335236 Free PMC article. Review.
-
Mechanical properties of ultrahigh-strength gold nanowires.Nat Mater. 2005 Jul;4(7):525-9. doi: 10.1038/nmat1403. Epub 2005 Jun 5. Nat Mater. 2005. PMID: 15937490
Cited by
-
Silver Nanowire Networks: Mechano-Electric Properties and Applications.Materials (Basel). 2019 Aug 8;12(16):2526. doi: 10.3390/ma12162526. Materials (Basel). 2019. PMID: 31398876 Free PMC article. Review.
-
Ruthenium complex based nanocomposite film with enhanced and selective electrochemical sensing of bifenthrin pesticide.RSC Adv. 2024 Sep 18;14(40):29542-29558. doi: 10.1039/d4ra04188g. eCollection 2024 Sep 12. RSC Adv. 2024. PMID: 39297048 Free PMC article.
-
Anisotropic Growth of Copper Nanorods Mediated by Cl- Ions.ACS Omega. 2022 Feb 16;7(8):7414-7420. doi: 10.1021/acsomega.2c00359. eCollection 2022 Mar 1. ACS Omega. 2022. PMID: 35252731 Free PMC article.
References
-
- Cahn JW, Lärché F.. Surface stress and the chemical equilibrium of small crystals-II. Solid particles embedded in a solid matrix. Acta Metall. 1982;30(1):51–56.
-
- Azizi A, Zou X, Ercius P, et al. Dislocation motion and grain boundary migration in two-dimensional tungsten disulphide. Nat Commun. 2014;5:4867. - PubMed
-
- Lee S, Im J, Yoo Y, et al. Reversible cyclic deformation mechanism of gold nanowires by twinning–detwinning transition evidenced from in situ TEM. Nat Commun. 2014;5:3033. - PubMed
-
- Wang YH, Xiong NN, Li ZL, et al. A comprehensive study of silver nanowires filled electrically conductive adhesives. J Mater Sci. 2015;26(10):7927–7935.
Publication types
LinkOut - more resources
Full Text Sources